This article was originally written in the Fall of 2010.
U.S. Department of Labor workforce projections for 2018 highlight that nine of the 10 fastest-growing occupations requiring at least a bachelor’s degree will necessitate significant scientific or mathematical training [1]. The United States’ science, technology, engineering, and math (STEM) workforce is aging while jobs requiring specialized training are growing at five times the rate of other occupations [1, 2]. STEM workers, who use science and math to solve problems, are needed to replace the many highly skilled workers who will retire over the next decade. A heterogeneous and culturally diverse workforce creates competitive advantage through greater creativity and innovation, and increased quality of team problem solving based on multiple perspectives [3-5]. Therefore, in order to sustain US capacity and increase global competitiveness for technological innovations, it is essential for people from a diverse representation of cultures, ages, and gender to enter STEM occupations.
Since 2000, women have earned approximately half of all science and engineering bachelor’s degrees[6]. However, further examination reveals that there is a significant gender gap in the number of women earning engineering degrees. Remaining nearly stagnant over the last fifteen years, women today represent only 18.6% of bachelor’s degrees awarded in engineering, and a diminutive 11% of the engineering workforce[6]. In order to effectively compete in the global marketplace, it is imperative that we advance the full and equitable participation of all Americans in science, engineering, and technology fields. The diverse viewpoints, approaches, and skills of women will benefit these high-tech industries, and in turn, positively affect our economy[3].
Aggressive and focused intervention efforts targeting women is recommended to address the gender gap in engineering[3]. While girls take more science and math classes and make better grades than boys, they are not readily choosing engineering as a college major and career path. Areas where consistent gender differences have emerged are children’s and adolescents’ interest in math and science, their beliefs about their abilities in math and science, and their perceptions of the importance of math and science for their futures[7]. Acknowledging and addressing these areas can increase girls’ awareness, interest, and confidence to pursue a career in engineering. Through an integrated approach to learning, engineering education has the potential to not only be a catalyst for improving K-12 STEM education[8], but to enable girls in their development towards a career choice in engineering. This increased investment in STEM education will boost U.S. global competitiveness by increasing productivity[3].
Review of the Literature
Achievement
On average, females receive higher grades in school in every subject including mathematics and science; females earn more credits in math and science courses than boys; and female high school graduates have a higher combined GPA in math and science courses than boys[9-12]. Despite this achievement, boys tend to outscore girls when tested on the same content in high-pressure situations, such as the SAT, NAEP, PISA and TIMSS exams[12-16]. While more females are participating in Advanced Placement mathematics and science, they are not performing at the levels of their male counterparts[17]. In 2009, 55 percent of AP test-takers were girls, but in STEM-related areas girls represent only 41% of test-takers[18]. In the same year, 38 percent of AP physics test-takers were girls, and girls made up only 19 percent of those taking AP computer science[19].
The foundations and explanations of gender differences in math skills remain unclear, as competing sides of the literature have yet been able to undeniably prove their positions on why female achievement in math and science trails behind males[17, 20]. It is suggested that girls will perform at the same level on high-stakes testing as their male classmates when they are encouraged to succeed, are given the necessary educational tools, and have visible female role models excelling in mathematics[21].
Awareness
In a nation that was seeded by freedom and opportunity, the roots of the United States of America are grounded by hard work, innovation, and determination. Most often through identification with a worker, ages 5 to 10 is the stage of life when the concept of working becomes ingrained in the child’s conception of his or her adult life[22]. Immersed in a society that is dominated and driven by work, and vulnerable to social influences of prestige and gender bias, children as young as five years of age begin to postulate what career they will one day have[23]. Young people tend to choose professions that are familiar[24], whether traditions in their family, or professions that have been exposed to them through education and experience. Young children can begin to gather information about careers and acquire the skills and competencies that will one day support success in the workplace[25].
A strong high school background, particularly in math, is “key to overall success in college” [26]. Pre-college courses that have the strongest impact on a student’s postsecondary education are high level mathematics courses in high school, according to Clifford Adelman: “Finishing a course beyond the level of Algebra II more than doubles the odds that a student who enters postsecondary education will complete a bachelor’s degree” [27]. Math skills are considered essential to success in STEM fields[19].
One out of every two middle school students indicate that they do not plan to take mathematics and science courses beyond what their schools require. Signaling a lack of awareness of course requirements for potential high-tech career trajectories, the same students indicate that they would be interested in going to college, and taking college-level mathematics courses[3]. This contrast heralds that students do not understand the importance of, and requirements for, taking rigorous mathematics and science courses in high school, pointing to the importance of encouraging exploration of a wide array of career trajectories so that students do not inadvertently build roadblocks to certain careers[28, 29].
Students generally have a poor understanding of what engineering is and what engineers do[30, 31], and females lack the role models necessary to envision themselves in this field[21, 32, 33]. Girls are attentive to the behaviors that women in their culture engage in and thus feel efficacious in and model those behaviors[21]. Girls’ attitudes regarding scientists and engineers have been influenced by the lack of female scientists in the media. With this absence of role models, many girls tend to view science and technology an unsuitable career choice and personally irrelevant to their lives. By concerted efforts to expose students to careers in science and engineering, these perceptions can be reversed[32]. Career awareness and having a sense of similarity to the people in a field is an important predictor of interest in that field[34].
Interest
About 80% of fourth graders report positive attitudes toward mathematics and science compared to an estimated 33% of eighth graders [35]. Despite gender similarities in achievement, boys reported more positive math attitudes and affect, and were more extrinsically and intrinsically motivated to do well in math than were girls [21, 36]. Studies show that students begin to lose interest in science, technology, engineering, and math by junior high school[3], and from early adolescence girls express less interest in science or math careers than boys[37-39]. A report by the Commission on the Advancement of Women and Minorities in Science, Engineering and Technology says that there are four points in life at which girls and women seem to lose interest in these subjects: as they enter middle school, late high school, college and graduate school, and in their professional lives[3].
A 2009 poll of 8-17 year-old students found 24 percent of boys but only 5 percent of girls showing interest in an engineering career[19]. Females are more likely than males to want to pursue a career in the area of health, or professional or managerial jobs, choosing occupations that place a greater emphasis on working with people and contributing to society[40-43]. Positive experiences in high school serve as the most consistent and important predictors of students future interest in science[44]. Girls are more likely to choose courses and careers in math and science if their interest in these fields is sparked and cultivated throughout the school years[45](as referenced by [7, 46]).
Confidence
Beginning at an early age, girls report less confidence in their math ability than boys do, even when no actual difference in math achievement exists[47, 48]. Fouad (2008) claims girls‘ feelings of self-confidence are a precursor to girls’ interests in science and math[49]. Students who lack confidence in skills they possess are less likely to engage in tasks in which those skills are required, and they will more quickly give up in the face of difficulty[50].
According to the Eccles et al. Expectancy Value Model, two of the key factors adolescents contemplate when making a decision are their self-concept of their abilities in an area and how much they value an area[51]. Simpkins & Davis-Kean found that differences in adolescents’ high school choices date back to their self-concepts and values in ninth grade, however, a high self-concept is the important determinant of course selection rather than the unique combination of high self-concept and high values[40]. Thus students with more confidence in their math and science abilities are more likely to excel in these subjects and pursue coursework and careers in these fields.
Gender stereotypes about gender roles and math may encourage girls to feel anxious and less confident[52]. Else-Quest (2010) postulates:
When girls develop in a societal context where women have careers in scientific research, they receive a clear message that STEM is within the realm of possibilities for them. Conversely, if girls’ mothers, aunts, and sisters do not have STEM careers, they will perceive that STEM is a male domain and thus feel anxious about math, lack the confidence to take challenging math courses, and underachieve on math tests. (p. 123)
Relating this to the expectancy-value model, Else-Quest’s theory supported by empirical data is: if a girl believes that the career opportunities available to or appropriate for women do not require mathematics skills, she is less likely to capitalize in the development of her mathematics skills, thus identifying math as less useful or valuable and believing she is not capable of doing math [21, 51, 53]. This theory provides a clear model for why cultural inequities and biased environmental factors in educational or career opportunities have an adverse impact on girls and women considering STEM careers[34].
Environmental factors
In a study engaging in the debate of biological versus environmental factors instigating the underrepresentation of women in STEM, it was found that men have higher mean TIMSS scores in all of the countries examined, but the size of these differences between men and women varies considerably, evincing the importance of environmental factors, whether parental, sociocultural, or educational[54, 55]. Strong implicit biases associated with gender and science influence early socialization and perpetuate gender stereotypes. These attitudes and messages skew girls’ academic pathways early, placing them on a trajectory which may limit future career options due to insufficient course foundations.
A recent comprehensive review of the research on sex differences in math showed evidence that children conceptualize mathematics and science as a “masculine” activities[11, 43]. This is not hard to imagine when 70% of more than half a million Implicit Association Tests completed by citizens of 34 countries revealed implicit stereotypes associating science with males more than with females[56]. In a study by Sadker and Sadker examining stereotyped perceptions, the most strongly endorsed gender-biased statement (for boys, girls, mothers, fathers, coaches, and teachers) was, “Men are naturally better at mathematics than women.” In contrast, the most strongly endorsed non-biased statement was, “It is just as appropriate for women to study mathematics as for men”[57]. Herein lies the social conflict: “women and men should have equal opportunities for success in math, even though men have more natural talent than women” [58] (p. 289). Implicit stereotypes and sex differences in science participation and performance are mutually reinforcing[56].
Unsupportive, biased classroom environments and outdated pedagogy inhibit women’s participation in STEM subjects[33]. Girls engage in science in a variety of ways[59], though some young women may refuse to participate in scientific activities that are incongruent with their gendered identities[60], seeking nontraditional ways to participate in science that are consistent with their gendered stereotypes[61]. Leedy found that even girls who are particularly motivated and talented in mathematics are not immune to the ill effects of gender bias, as they too experienced decreased confidence in math[58]. Schools disrupt female math trajectories by institutionalizing gendered expectations that work to discourage girls’ pursuit of math-related skills[57]. Consequently, popular literature that emphasizes gender differences may in fact reinforce stereotypes that girls lack mathematical and scientific aptitude[62]. Environmental factors are at work, and gender equity in education is important not only for girls’ math achievement but also for girls’ self-confidence and valuing of mathematics[21, 50].
Integrated Science and Math Education
There are several practices that promote an equitable learning environment for girls and have a positive impact on their continuation in quantitative disciplines such as engineering. They include collaborative learning, hands-on experiences, engaging authentic activities, an emphasis on practical applications, and the teaching of science and math in a more holistic, inclusive and social context [26, 33, 63-65].
Labs, field trips, and career counseling, enhance girls’ performance in scientific subjects, allowing them to see the relevance of science and mathematics in the broader context of work and life [66]. In addition, cooperative learning groups and active learning motivate young women to study mathematics and science [67, 68]. NAEP mathematics scores were higher for eighth graders who participated in hands-on learning activities than for those who did not [63]. However, cooperative learning and hands-on activities should be monitored by the instructor. Some research has documented situations where girls were less likely to receive help from boys in cooperative groups, and, if there is only one girl in a group, the boys usually ignore her [69]. Moreover, boys are more likely to dominate resources in small, coed settings, while girls were more often passive participants[70, 71]. By highlighting broader, multidisciplinary and collaborative applications early in a curriculum, as opposed to first focusing on technical aspects, women and men are more likely to report more interest in a program and not quit[19].
When integrated with science, math provides opportunity for students to apply the discipline to real and relevant situations, a way that develops depth rather than breadth in a mathematics curriculum[72]. NAEP mathematics scores were higher for 17-year-olds with access to computers to learn mathematics and solve mathematical problems[73], an example of how an integrated approach enhanced mathematical learning. The integration of real-world interdisciplinary problems with school subject matter can support students’ efforts in constructing patterns and meaningful knowledge, via the identification of math and science as relevant components of their world[72, 74].
Haussler and Hoffman developed a new cooperative physics curriculum based on data from a student interest study[75]. The seven guidelines for stimulating interest in physics among girls (and in boys) is an approach of teaching physics that: provides opportunities to marvel; links content to prior experiences for both boys and girls; provides first-hand experiences; encourages discussions and reflections on the social importance of physics; connects physics with applications; shows physics in relation to the human body; and demonstrates the benefit and use of treating physics quantitatively[76]. Haussler and Hoffman found that adapting the curriculum to the interests of girls was also advantageous for boys. This method is a highly integrated approach of teaching physics, which would benefit from engineering activities focused on inquiry and design.
Integration uses examples, data, and information from a variety of disciplines to illustrate the key concepts, principles, generalizations, and theories in a subject area or discipline[77]. Davison et al. (1995) suggests five types of science and mathematics integration that can be used in interdisciplinary curriculum development: discipline specific, content specific, process, methodological, and thematic[72]. Each of these forms of integration is described in Table 1. These methods should not be considered mutually exclusive, but instead a framework for integrating science and math. Engineering lessons and activities can be an active catalyst for each of these methods, thus improving K-12 STEM education[8].
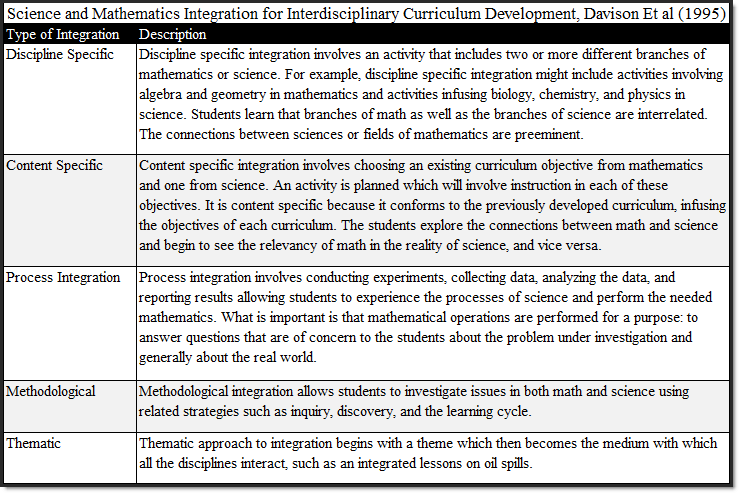
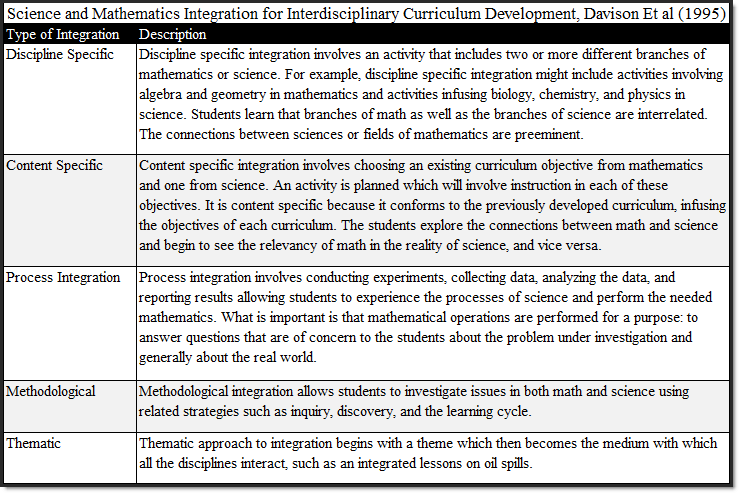
Engineering Education
Engineering has strong connections to many other disciplines, particularly mathematics and science. Engineers employ scientific knowledge and mathematic principles in their work, and scientists and mathematicians benefit from the products of engineering, known as technology. Engineers exercise mathematics to describe and analyze data and to develop models for evaluating design solutions. It is imperative for engineers to be knowledgeable about the science that is relevant to the problem they are engaged in solving. It is not uncommon for research conducted by engineers to result in new scientific discoveries[8].
Students need to be taught the principles of engineering and be given positive experiences that may encourage them to pursue an engineering career[78]. Greater knowledge of occupational specialties is associated with greater confidence in choice[79, 80]. Engineering education needs to begin in elementary school while student interest in mathematics and science is still high, however integrating engineering concepts, practicing related skills, and exploring associated careers in any classroom has the potential to increase the number of students who pursue engineering[81]. In addition, as was noted in Engineering in K–12 Education: Understanding the Status and Improving the Prospects, the potential positive impact of engineering on the learning of mathematics and science is tremendous[8]. K-12 engineering education should emphasize design, incorporate important and developmentally appropriate mathematics, science, and technology knowledge and skills, and should promote engineering habits of mind[78].
Team-based design satisfies hands-on learning curriculum, integrating math and science fundamentals through creative, self-directed learning. Open ended and multidisciplinary, design provides context for theoretical foundation concepts, and forces effective teamwork skills. Designing within constraints and through iteration unleashes creativity and motivates deeper understanding[82].
The National Academy of Engineering (NAE) publication Technically Speaking emphasizes the need for all people to obtain technological literacy to function in the modern world[83]. K–12 STEM education contributes to scientific and technological literacy, which are important attributes for all citizens[8]. Research from engineering education and the learning sciences suggests that students need to develop a deep understanding of fundamental science, mathematics and technology principles across P-12 if they want to pursue a wide range of engineering and technical career opportunities[84].
Strategies that work
While girls are plagued by numerous elements that seem to discourage a career in engineering, research positively suggests that there are ways to increase girls’ interest in the field[37]. It is important to continue to transform the image of the STEM professions and their practitioners so that the image is positive and inclusive for women[3]. A study by Halpern et al. (2007) identifies five strategies to encourage females in mathematics in science, including: teaching females students that success in mathematics and science is not based on innate ability, increasing exposure of female students to successful female mathematicians and scientists, providing “prescriptive, informational feedback,” creating classroom environments that engage and create lasting interest in science and math, and providing additional training for female students in spatial skills[7] (p. 6). Programs for girls that combine hands-on activities, role models, mentoring, internships, and career exploration have improved girls’ self-confidence and interest in STEM courses and careers and helped reduce sexist attitudes about STEM [68, 85].
Recommendation
In order to meet the forecasted demands[86] for U.S. Labor in technical areas that require scientific and mathematical training, it is imperative that educators and students begin to understand STEM professions and the role of engineers. For students to make informed career decisions, it is essential for counselors to provide accurate STEM career information and be equipped to provide guidance on how students can prepare for such careers.
Engineering is essential to our health, happiness & safety. Engineers are creative & collaborative problem-solvers who make a world of difference and help shape the future. These are the messages that educators can use to promote student awareness of and interest in engineering. By advocating science and math in a more holistic, inclusive and social context, more students will develop confidence in these subjects and be prepared to pursue a career in engineering.
References
1. National Science Board, Science and engineering indicators 2010 (NSB 10-01). 2010, National Science Foundation: Arlington, VA.
2. American Association of University Women, Improve Girls’ and Women’s Opportunities in Science, Technology, Engineering, and Math. 2010, American Association of University Women.
3. Congressional Commission on the Advancement of Women and Minorities in Science Engineering and Technology Development. Land of Plenty: Diversity as America’s Competitive Edge in Science, Engineering and Technology. 2000 12 November 2010; Available from: http://www.nsf.gov/pubs/2000/cawmset0409/cawmset_0409.pdf.
4. Robinson, G. and K. Dechant, Building a Business Case for Diversity. Academy of Management Executive, 1997. 11: p. 21-31.
5. American Management Association (AMA), Senior Management Teams: Profiles and Performance. 1998, New York, NY: American Management Association.
6. NSF. NSF Women, Minorities, and Persons with Disabilities in Science and Engineering Report. 2007; Available from: http://www.nsf.gov/statistics/wmpd/start.htm.
7. Halpern, D., et al., Encouraging Girls in Math and Science. IES Practice Guide. NCER 2007-2003. National Center for Education Research, 2007: p. 55.
8. Committee on Standards for K-12 Engineering Education, Standards for K-12 Engineering Education? 2010, National Academy of Sciences: Washington, DC.
9. Dwyer, C. and L. Johnson, Grades, accomplishments, and correlates, in Gender and fair assessment, W.W. Willingham and N.S. Cole, Editors. 1997, Erlbaum: Mahwah, NJ. p. 127–156.
10. Kimball, M.M., A new perspective on women’s math achievement. Psychological Bulletin, 1989. 105: p. 198–214.
11. Shettle, C., et al., The nation’s report card: America’s high school graduates, in Washington, DC: US Government Printing Office. 2007.
12. U.S. Department of Education – National Center for Education Statistics. 2005 NAEP High School Transcript Study. February 2010 12 November 2010]; Available from: http://nces.ed.gov/nationsreportcard/hsts/.
13. Gonzales, P., et al., Highlights From TIMSS 2007: Mathematics and Science Achievement of U.S. Fourthand Eighth-Grade Students in an International Context (NCES 2009-001). 2009, U.S. Department of Education.
14. U.S. Department of Education – National Center for Education Statistics. The Nation’s Report Card: America’s High School Graduates (NCES 2007-467). 2007 12 November 2010]; Available from: http://nces.ed.gov/nationsreportcard/pdf/studies/2007467_4.pdf.
15. College Board. College-Bound Seniors 2010 Total Group Profile Report: SAT. 2010 11/24/2010]; Available from: http://professionals.collegeboard.com/profdownload/2010-total-group-profile-report-cbs.pdf.
16. Organisation for Economic Co-operation and Development. PISA Database: Interactive Data Selection (United States by Sex). 2006 11/24/2010]; Available from: http://pisa2006.acer.edu.au/interactive.php.
17. Campbell, D.M. and et. al., Trends in Advanced Placement Science and Mathematics Test-Taking Among Female Students in California: A Latent Variable Approach. Electronic Journal of Science Education, 2009. 13(2): p. 62-82.
18. College Board. Program Summary Report. 2009; Available from: http://professionals.collegeboard.com/profdownload/program-summary-report-09.pdf.
19. Hill, C., C. Corbett, and A. St Rose, Why So Few? Women in Science, Technology, Engineering, and Mathematics. American Association of University Women, 2010: p. 134.
20. Gibbs, B.G., Reversing fortunes or content change? Gender gaps in math-related skill throughout childhood. Social Science Research, 2010. 39(4): p. 540-569.
21. Else-Quest, N.M., J.S. Hyde, and M.C. Linn, Cross-national patterns of gender differences in mathematics: A meta-analysis. Psychological Bulletin, 2010. 136(1): p. 103-127.
22. Havighurst, R., Youth in exploration and man emergent, in Man in a world at work, H. Borow, Editor. 1964, Houghton Mifflin: Boston. p. 215–236.
23. Gottfredson, L., Circumscription and compromise: A developmental theory of occupational aspirations. Journal of Counseling Psychology, 1981. 28(6): p. 545-579.
24. Parker, W. and J. Jarolimek, Social studies in elementary education. 1997: Prentice-Hall, Inc., Simon and Schuster/A Viacom Company, Upper Saddle River, New Jersey 07458.
25. Duffy, P., Skills for Life. Fitting Career Awareness into the Curriculum. Instructor, 1989. 98(6): p. 36-38.
26. Campbell, P., et al., Upping the Numbers: Using Research-Based Decision Making To Increase Diversity in the Quantitative Disciplines. 2002, GE Fund: Fairfield, CT.
27. Adelman, C., Answers in the Toolbox: Academic Intensity, Attendance Patterns, and Bachelor’s Degree Attainment (PLLI 1999–8021). US Department of Education. Washington, DC: Office of Educational Research and Improvement, 1999.
28. White Paper prepared for U. S. Secretary of Education Richard W. Riley. Math Equals Opportunity. 1997 12 November 2010]; Available from: www2.ed.gov/pubs/math/mathemat.pdf.
29. Rudasill, K.M. and C.M. Callahan, Academic Self- Perceptions of Ability and Course Planning Among Academically Advanced Students. Journal of Advanced Academics, 2010. 21(2): p. 300-329.
30. Cunningham, C., C. Lachapelle, and A. Lindgren-Streicher, Assessing elementary school students’ conceptions of engineering and technology. American Society of Engineering Education, Portland, OR, 2005.
31. Knight, M. and C. Cunningham. Draw an engineer test (DAET): Development of a tool to investigate students’ ideas about engineers and engineering. in American Society for Engineering Education. 2004. Salt Lake City, UT.
32. Huber, R. and G. Burton, What do students think scientists look like? School Science and Mathematics, 1995. 95(7): p. 371-376.
33. Fancsali, C. What We Know About Girls, STEM and Afterschool Programs. 2002 12 November 2010]; Available from: www.jhuapl.edu/mesa/resources/docs/whatweknow.pdf.
34. Cheryan, S. and V. Plaut, Explaining Underrepresentation: A Theory of Precluded Interest. Sex Roles, 2010. 63(7): p. 475-488.
35. Sherman, J., S. Honegger, and J. McGivern, Comparative Indicators of Education in the United States and Other G-8 Countries: 2002 (NCES 2003–026), in National Center for Educational Statistics, U.S.D.o. Education, Editor. 2003.
36. Hyde, J.S., et al., Gender Comparisons of Mathematics Attitutudes and Affect. Psychology of Women Quarterly, 1990. 14(3): p. 299.
37. Turner, S.L. and R.T. Lapan, Evaluation of an intervention to increase non-traditional career interests and career-related self-efficacy among middle-school adolescents. Journal of Vocational Behavior, 2005. 66(3): p. 516-531.
38. Lapan, R., et al., Seventh graders’ vocational interest and efficacy expectation patterns. Journal of Career Development, 2000. 26(3): p. 215-229.
39. Turner, S.L., et al., Gender Differences in Holland Vocational Personality Types: Implications for School Counselors. Professional School Counseling, 2008. 11(5): p. 317-326.
40. Simpkins, S.D. and P.E. Davis-Kean, The intersection between self-concepts and values: Links between beliefs and choices in high school. New Directions for Child and Adolescent Development, 2005. 2005(110): p. 31-47.
41. Ashby, J.S. and I. Schoon, Career success: The role of teenage career aspirations, ambition value and gender in predicting adult social status and earnings. Journal of Vocational Behavior, 2010. 77(3): p. 350-360.
42. Duffy, R. and W. Sedlacek, What is most important to students’ long-term career choices: analyzing 10-year trends and group differences. Journal of Career Development, 2009. 34(2): p. 149-163.
43. Jones, M., A. Howe, and M. Rua, Gender differences in students’ experiences, interests, and attitudes toward science and scientists. Science Education, 2000. 84(2): p. 180-192.
44. Tolley, K., The science education of American girls: A historical perspective. 2003, London: RoutledgeFarmer.
45. Wigfield, A., et al., Development of achievement motivation, in Handbook of child psychology, N. Eisenberg, Editor. 2006, Wiley: New York.
46. Page, M.C., L.E. Bailey, and J. Van Delinder, The Blue Blazer Club: Masculine Hegemony in Science, Technology, Engineering, and Math Fields. Forum on Public Policy Online, 2009.
47. Herbert, J. and D. Stipek, The emergence of gender differences in children’s perceptions of their academic competence. Journal of Applied Developmental Psychology, 2005. 26(3): p. 276-295.
48. Pajares, F., ed. Gender Differences in mathematics self-efficacy beliefs. Gender differences in mathematics: An integrative psychological approach ed. A.M.G.J.C. Kaufman. 2005, Cambridge University Press: Boston. 294–315.
49. Fouad, N. Tracking the reasons many girls avoid science and math. 2008 [cited 2010 November 21]; Available from: http://www.eurekalert.org/pub_releases/2008-09/uow–ttr090508.php.
50. Pajares, F., Self-Efficacy Beliefs and Mathematical Problem-Solving of Gifted Students. Contemporary Educational Psychology, 1996. 21(4): p. 325-344.
51. Eccles, J.S., Understanding women’s educational and occupational choices. Psychology of Women Quarterly, 1994. 18(4): p. 585.
52. Steele, C.M., A threat in the air: How stereotypes shape intellectual identity and performance. American Psychologist, 1997. 52(6): p. 613-629.
53. Frome, P.M. and J.S. Eccles, Parents’ influence on children’s achievement-related perceptions. Journal of Personality and Social Psychology, 1998. 74(2): p. 435-452.
54. Penner, Andrew M., Gender Differences in Extreme Mathematical Achievement: An International Perspective on Biological and Social Factors. American Journal of Sociology, 2008. 114(S1): p. S138-S170.
55. Andreescu, T., et al., Cross-cultural analysis of students with exceptional talent in mathematical problem solving. Notices of the American Mathematical Society, 2008. 55(10): p. 1248–60.
56. Nosek, B., et al., National differences in gender–science stereotypes predict national sex differences in science and math achievement. Proceedings of the National Academy of Sciences, 2009. 106(26): p. 10593.
57. Sadker, M. and D. Sadker, Failing at fairness: How America’s schools cheat girls. 1994, New York: Touchstone.
58. Leedy, M., D. LaLonde, and K. Runk, Gender equity in mathematics: Beliefs of students, parents, and teachers. School Science and Mathematics, 2003. 103(6): p. 285-292.
59. Brickhouse, N.W., P. Lowery, and K. Schultz, What Kind of a Girl Does Science? The Construction of School Science Identities. Journal of Research in Science Teaching, 2000. 37(5): p. 441-458.
60. Carlone, H. Identifying and expanding the meaning of ‘‘scientist’’in school science: Implications for the participation of girls. in annual meeting of the American Educational Research Association. 1999. Montreal, Quebec.
61. Eisenhart, M. and E. Finkel, Women’s Science. 1998, Chicago: University of Chicago Press.
62. Hyde, J. and M. Linn, Gender similarities in mathematics and science. SCIENCE, 2006. 314(5799): p. 599.
63. Wenglinsky, H., How Teaching Matters: Bringing the Classroom Back into Discussions of Teacher Quality. Educational Testing Service, Princeton, NJ. Policy Information Center, 2000.
64. Barton, A.C., Feminist Liberatory Science Education?, in Feminist Science Education. 1998, Teacher’s College Press: New York, NY. p. 1-19.
65. Carlson, L. and J. Sullivan, Hands-on engineering: learning by doing in the integrated teaching and learning program. International Journal of Engineering Education, 1999. 15: p. 20-31.
66. Kahle, J., Opportunities and obstacles: Science education in the schools, in The equity equation: Fostering the advancement of women in the sciences, mathematics, and engineering. 1996, Jossey-Bass. p. 57-95.
67. Bartsch, I., E. Snow, and S. Bell, FLEDGE-ling: A Science Program for Girls 1998. 4(4): p. 321-331.
68. Ferreira, M.M., The Effects of an After-School Science Program on Middle School Female Students’ Attitudes towards Science, Mathematics and Engineering. 2001.
69. Lee, V., Gender equity and the organization of schools, in Gender, equity, and schooling: Policy and practice. 1997, Routledge. p. 135–158.
70. Jovanovic, J. and S. King, Boys and Girls in the Performance-Based Science classroom: Who’s doing the performing? American Educational Research Journal, 1998. 35(3): p. 477.
71. Volman, M. and E. van Eck, Gender equity and information technology in education: The second decade. Review of Educational Research, 2001. 71(4): p. 613.
72. Davison, D., K. Miller, and D. Metheny, What does integration of science and mathematics really mean? School Science and Mathematics, 1995. 95(5): p. 226-230.
73. Campbell, J., C. Hombo, and J. Mazzeo, NAEP 1999 Trends in Academic Progress: Three Decades of Student Performance. 2000: ED Pubs, PO Box 1398, Jessup, MD 20794-1398. Tel: 877-433-7827 (Toll Free). US Government Printing Office, Superintendent of Documents, Mail Stop: SSOP, Washington, DC 20402-9328.
74. Roth, W., Problem-centered learning for the integration of mathematics and science in a constructivist laboratory: A case study. School Science and Mathematics, 1993. 93(3): p. 113-122.
75. Häussler, P. and L. Hoffmann, A curricular frame for physics education: Development, comparison with students’ interests, and impact on students’ achievement and self-concept. Science Education, 2000. 84(6): p. 689-705.
76. Häussler, P. and L. Hoffmann, An intervention study to enhance girls’ interest, self concept, and achievement in physics classes. Journal of Research in Science Teaching, 2002. 39(9): p. 870-888.
77. Banks, J., Multicultural education: Development, dimensions, and challenges. Phi Delta Kappan, 1993. 75(1).
78. Katehi, L., G. Pearson, and M. Feder, Engineering in K-12 education: Understanding the status and improving the prospects. 2009: National Academies Press.
79. Shivy, V.A. and T.N. Sullivan, Engineering students’ perceptions of engineering specialties. Journal of Vocational Behavior, 2005. 67(1): p. 87-101.
80. Lent, R.W., S.B. Brown, and G. Hackett, Social cognitive career theory, in Career Choice and Development, D. Brown and Associates, Editors. 2002, Erlbaum: Hillsdale, NJ. p. 255–311.
81. Strutz, M. A Retrospective study of the elementary-school experiences, influences, skills, and traits of talented engineers (AC 2009-693). in American Society for Engineering Education Annual Conference. 2009. Austin, Texas.
82. Carlson, L. and J. Sullivan, Exploiting Design to Inspire Interest in Engineering Across the K-16 Engineering Curriculum. International Journal of Engineering Education, 2004. 20(3): p. 372-378.
83. Pearson, G. and A. Young, Technically speaking: Why all Americans need to know more about technology. 2002: National Academies Press.
84. Brophy, S., et. al., Advancing Engineering Education in P-12 Classrooms [Part of the special issue, Educating Future Engineers: Who, What, and How]. Journal of Engineering Education, 2008. 97(3): p. 369-87.
85. Campbell, P. and K. Steinbrueck, Striving for gender equity: National programs to increase student engagement with math and science. Washington, DC: American Association for the Advancement of Science, 1996.
86. Bureau of Labor Statistics Employment Projections by Occupation.